Nano compartments may aid drug delivery, catalyst design
By Bill Steele
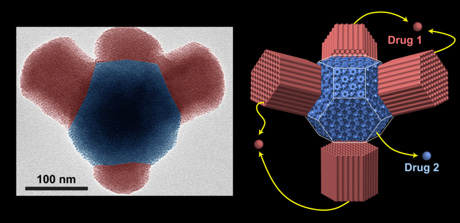
Spongelike nanoparticles whose pores can be filled with drugs offer the promise of drug delivery to specific targets in the body, avoiding unpleasant side effects. Cornell researchers have now created nanoparticles with separate compartments that could carry two or more different drugs to the same location, with precise control over the amounts. The technology might also be applied to catalysts used to enhance chemical reactions, which are sometimes formed into porous nanoparticles to expose more surface area; compartmented particles could allow two or more catalysts to work in sequence.
Ulrich Wiesner, the Spencer T. Olin Professor of Materials Science and Engineering, and first authors, Cornell researcher Teeraporn Suteewong and graduate student Hiroaki Sai, have tweaked familiar “sol-gel” chemistry used to self-assemble porous silica particles, making the assembly shift gears partway through to create what amounts to two or more different nanoparticles joined together, while controlling how one particle grows out of another, a process referred to as epitaxial growth.
“It’s the first time I’m aware of that the shapes of porous silica nanoparticles have been controlled via epitaxy,” Wiesner said. “The cubic lattice and the hexagonal lattice have a well-defined relationship.” The products so far are fairly simple particles with two or three compartments, but the methods might be extended to create much more complex structures, he said.
The discovery was partly serendipitous. While making ordinary nanoparticles, the scientists saw a small fraction with hexagonally structured porous branches growing out of a cubic core particle. “We set out to understand what controls that,” Wiesner said.
Wiesner’s team and researchers from Memorial Sloan Kettering Cancer Center in New York City report their results in the April 19 issue of the journal Science as “Multicompartment Mesoporous Silica Nanoparticles with Branched Shapes: An Epitaxial Growth Mechanism.” The other researchers include graduate student Robert Hovden; David Muller, professor of applied and engineering physics; Sol M. Gruner, professor of physics; and Michelle Bradbury, M.D., Ph.D., Memorial Sloan-Kettering Cancer Center.
The starter for the process is a mixture of organosilanes, molecules built around carbon and silicon atoms, and surfactants. Surfactants, of which the prime example is soap, have one end that likes water and another “oily” end that tries to stay away from it. So in water surfactants form micelles, tiny spherical bundles with the water-loving end out and the oily part tucked away in the center. In the sol-gel process the micelles act as cages around which silica from the orgaosilanes forms, building particles about a hundred nanometers in diameter. When the micelles are washed away what remains is a porous silica structure with pores two to three nanometers in size. “The micelles are placeholders for the pores,” Wiesner explained. (A nanometer is a billionth of a meter, about the length of three atoms in a row.)
The type of pore lattice depends, among other things, on the pH, or acidity, of the solution. The researchers added ethyl acetate, a chemical that breaks down in water and in the process makes the solution more acidic, to act as a timer to change the output of the reaction partway through. At first a cubic lattice forms, building cubical particles. As acidity increases the reaction path changes to make a hexagonal lattice creating cylinders that begin to grow out of the faces of the cubes. The number of cylinders and their length can be controlled by the concentration of ethyl acetate, generating tripods or even tetrapods.
“Previous work in my group and that of others has focused on how to control the pore structure,“ Wiesner said. “Here we use the pore structure to control the shape of the nanoparticles.”
In a hint of the future, the researchers were able to connect two or three cubes with cylindrical bridges between them, perhaps the beginning of a network of cubes and tubes like a nanoscale hamster habitat. “We have learned to switch the growth conditions. If we can switch back we might be able to grow all sorts of funky architectures,” Wiesner said.
The National Science Foundation supported this research.
This story was updated April 18, 2013.
Media Contact
Get Cornell news delivered right to your inbox.
Subscribe