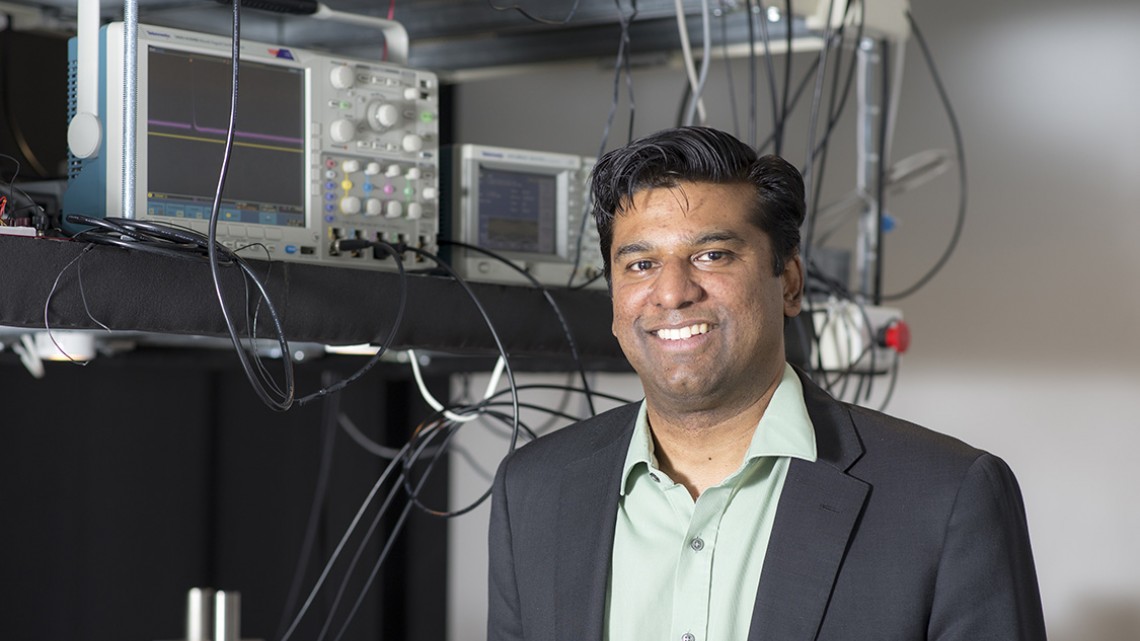
Steven Adie is pictured in his lab.
New microscopy method could benefit study of migrating cancer cells
By Tom Fleischman
More than 30 years ago, Bell Laboratories scientist Arthur Ashkin, Ph.D. ’52, developed a method for using tightly focused laser light to manipulate microparticles. That method, originally called “single-beam gradient force optical trap,” became known by a more descriptive moniker: “optical tweezers.”
That method was the product of work Ashkin – now 95 years old and the holder of 47 patents – did more than 15 years earlier involving microwaves, nonlinear optics and laser trapping. And it’s that earlier work, involving less-focused laser light, that has inspired a new method from the lab of Steven Adie.
Adie, assistant professor in the Meinig School of Biomedical Engineering, has come up with a way to use pressure from pulses of laser light to create sub-nanometer shifting of micron-sized particles embedded in soft tissue-like media. The displacement of the particles is measured by a second beam in a process called photonic-force optical coherence elastography (PF-OCE).
This technique has the potential to change how biomedical scientists study the relationship between cells and their environment, particularly when using engineered tissue cultures such as organoids.
Doctoral student Nichaluk Leartprapun, from the Adie Lab, is lead author of “Photonic Force Optical Coherence Elastography for Three-dimensional Mechanical Microscopy,” published May 25 in Nature Communications.
The good thing about optical tweezers is they offer researchers the ability to control a single particle, or bead, with a highly focused beam of laser light. But that’s also what limits the light’s use in studying the local mechanical properties at each bead’s location in 3D. “Practically, it takes hours to [study particles in] a 2D plane,” Adie said, “and if you were to do a full 3D volume like we’re doing, it could take days.”
A weakly focused beam, on the other hand, can apply force over a more generalized area and over an extended depth range. The beam “jiggles the beads up and down,” Adie said, and their displacement values are measured using optical coherence tomography – a second laser beam – giving the researchers an idea of the local mechanical properties of the medium surrounding each bead.
Adie’s team used optical radiation pressure from a weakly focused beam to “push” on beads embedded in a hydrogel, and isolate the movement of each bead via PF-OCE. This unveils the mechanical response from a large number of randomly distributed beads in 3D.
The technique also allows for time-lapse 3D mechanical microscopy, something that was not possible using traditional methods such as atomic force microscopy, which is only useful for interrogating the 2D surface of a material.
“There’s a lot of work currently in the field of mechanobiology,” Adie said. “For this, 3D environments are really necessary in order to properly study the biological behavior and role of mechanical interactions between cells and their microenvironment.”
One major stumbling block in Adie’s work on PF-OCE: The photothermal (absorption-mediated heating) response of the hydrogel to the laser light drowned out the signal they were seeking, which was the bead displacement. “It took us a fair bit of time to solve it, but we got there,” he said.
This technique, Adie said, will be useful in studying the effect cells – including migrating cancer cells – have on their environment. “As cells migrate, they end up applying forces to the environment that they’re in, and those forces actually change the mechanical properties [of the environment],” Adie said. “There’s an active stiffness of the medium that we would like to be able to probe.”
Future work will involve taking measurements using smaller laser wavelengths, which will minimize the interference caused by the hydrogel’s photothermal response.
Adie’s group last year obtained a patent for this two-beam PF-OCE technique.
Other contributors included Rishyashring Iyer, M.Eng. ’17; Gavrielle Untracht ’11, M.S. ’16 (engineering physics); and doctoral student Jeffrey Mulligan, all from the Adie Lab.
Support for this work came from the National Institutes of Health, and from Adie’s Cornell Discovery and Innovation Research Seed Award. This work made use of the Cornell Center for Materials Research, which is supported by the National Science Foundation.
Media Contact
Get Cornell news delivered right to your inbox.
Subscribe