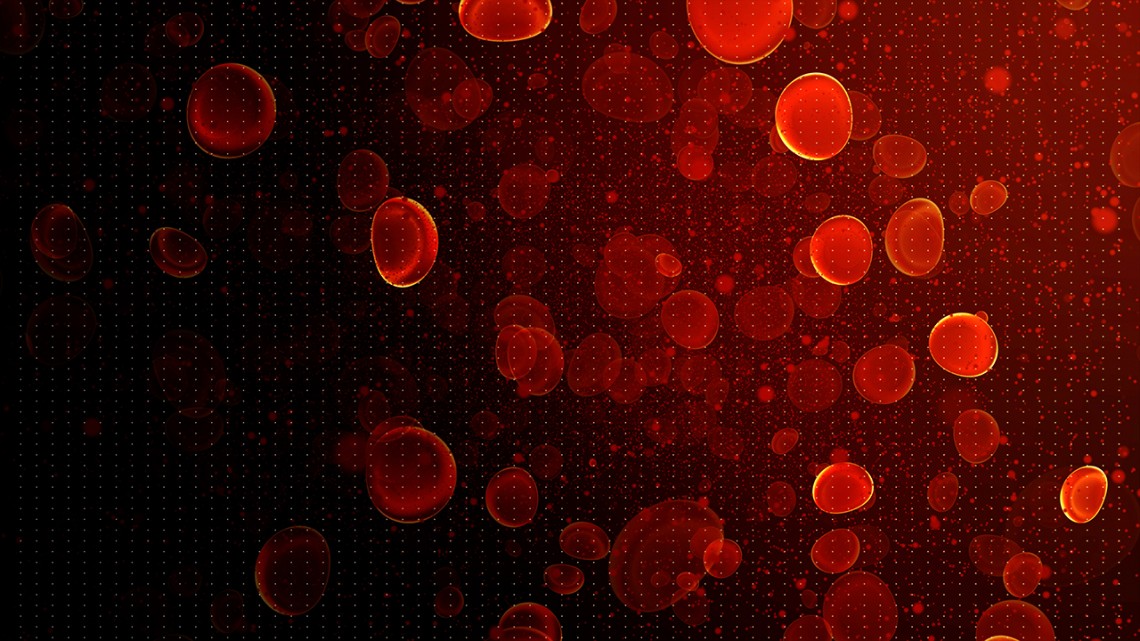
Researchers led by Nicholas Abbott, a Tisch University Professor in the Robert F. Smith School of Chemical and Biomolecular Engineering, created a way of using synthetic liquid crystals to squeeze red blood cells and gain new insight into individual cells’ mechanical properties.
Liquid crystals give red blood cells mechanical squeeze
By David Nutt
Red blood cells are not only diligent workers, delivering oxygen from the lungs to the rest of the body – they are also incredibly flexible. Their soft, pliable construction enables them to bend and twist to fit through billions of tiny, cramped capillaries.
At least that’s the case when they’re functioning correctly. In some diseases, such as sickle cell anemia and malaria, the elasticity and mechanical properties of red blood cells are compromised.
Scientists hoping to understand these diseases can take heart: Researchers led by Nicholas Abbott, a Tisch University Professor in the Robert F. Smith School of Chemical and Biomolecular Engineering, created a way of using synthetic liquid crystals to squeeze red blood cells and gain new insight into individual cells’ mechanical properties. The process also illuminates minuscule differences between cells within a large population as each cell reacts to the same strain or force, revealing just how diverse the cells are.
The group’s paper, “Dynamic and Reversible Shape-Response of Red Blood Cells in Synthetic Liquid Crystals,” published Oct. 2 in Proceedings of the National Academy of Sciences. The lead author is former postdoctoral researcher Karthik Nayani, now an assistant professor at University of Arkansas.
“There are ways of characterizing red blood cells by putting a bunch of red blood cells in a beaker and basically stirring it,” Abbott said. “That ultimately says something about the mechanical properties of all the cells in the beaker, but it doesn’t tell you about which 10 are rigid and will block your capillaries, and the other 10,000 that are just fine. But if you can measure mechanical properties at the single-cell level, you can say something about the outliers in the population. And often it’s the outliers which caused the problem.”
Abbott’s research group specializes in studying the interactions of molecules, the emergence of hierarchies of molecular organization, and their effect on mechanical and optical properties. The team has been particularly interested in the opportunities presented by synthetic liquid crystals, often described as the “fifth phase of matter.” Liquid crystals flow like a liquid but have the internal organization and long-range atomic order of a solid crystal. As synthetic materials, they have the potential to provide new functions when interacting with complex biological systems, like mammalian cells.
The creation of liquid crystals is itself a complex process, requiring just the right balance of concentrations and compositions to find the narrow window between other phases, such as crystals and liquids.
To put the squeeze on red blood cells, Abbott’s team used a type of liquid crystal known as a chromonic phase, formed from aqueous solutions of disodium cromoglycate (DSCG). This liquid crystal was originally developed as a treatment for asthma. Other compounds belonging to this class of liquid crystals are commonly used as food dyes, such as those responsible for giving Doritos their distinctive orange hue.
Unlike other liquid crystals, chromonics can host living mammalian cells without destroying the cell membrane, therefore making the material compatible with biological systems.
The researchers solved an additional challenge in establishing the right balance of osmotic pressure inside the red blood cell – filled as it is with proteins, such as hemoglobin, and other small molecules – and the liquid crystal’s non-physiological environment. If the pressure is not perfectly balanced, water will either flow across the membrane and into the red blood cell, swelling it until it bursts, or flow out and cause it to shrink.
“Initially, we saw the red blood cells swell and pop,” Abbott said. “Then we started tinkering with the composition of the liquid crystal and noticed that the lifetime of the red blood cell was increasing. And so we kept working in that direction.”
Once the osmotic balance had been achieved, the researchers began to manipulate the forces exerted by the liquid crystal on the cells by increasing the temperature and fine-tuning the concentration. The mechanical stress caused the red blood cells’ natural shape – which is a biconcave disc with a hollow depression in the center, similar to a breath mint – to stretch and deform.
Surprisingly, the red blood cells folded in different ways and displayed varying degrees of stiffness. Although the cells look alike before being placed into the liquid crystal, the liquid crystal reveals them to be highly variable in their mechanical properties.
“There’s a lot of heterogeneity within the population,” Abbott said. “If you want to understand the origins of disease, and you want to understand the effects of biological agents on cells, you have to consider that not every cell is the same as the one next to it.”
This imaging process can be used to analyze thousands of red blood cells within minutes, collecting data on the overall population and individual cells simultaneously. The method can also be applied to other cell types, and to differentiate between healthy and diseased cells.
Co-authors include researchers from the University of Wisconsin.
The research was supported by the National Science Foundation and the Army Research Office.
Media Contact
Get Cornell news delivered right to your inbox.
Subscribe